Dismantling the Royal Society Large-Scale Electricity Storage Report
The Royal Society report makes extraordinary claims that do not stand up to scrutiny.
Extraordinary claims require extraordinary evidence. Carl Sagan
Summary
The Royal Society (RS) has recently released its Large-Scale Electricity Storage report that says we can provide the electricity we need using wind and solar power supported by large-scale hydrogen storage. The report makes some extraordinary claims that are interrogated by this report that also seeks to find the extraordinary evidence required to validate their claims. One positive aspect of the RS report is the painstaking analysis of the variations in wind and solar power we might expect over yearly and decadal timescales that drive the need for a very large energy store. The RS report also effectively rules out batteries as a viable alternative as a large-scale energy store.
However, the positive parts of the report stop there. They begin by assuming that electricity demand will be 570TWh in 2050 which represents roughly halving the energy demand across residential, transport and industrial and commercial categories. The evidence from Our World in Data shows that rich economies require high energy consumption to thrive. There are no rich countries with low energy consumption and those countries that have reduced energy consumption have grown more slowly, or even shrunk. The first extraordinary claim of low energy consumption fails because the evidence shows that if we allow that to happen, we will be much poorer.
The report then goes on to assume that the profile of electricity demand will be the same as today. However, as we move from gas to electricity to heat our homes and offices, the winter surge in electricity demand will be further exaggerated. Moreover, demand will change from year to year such as during the cold winter in 2010 that also coincided with a calm period when we would have generated much less renewable electricity. These variations in demand profile will lead to more generation capacity and an even bigger energy store than RS assumes, pushing up costs.
On the supply-side, the report assumes unrealistic load factors for both onshore and offshore wind. They assume that the installed capacity in 2050 will have load factors far higher than has been achieved so far. Even allowing for some technological improvements, the capacity they need to meet their low generation target will need to increase by at least 20%. This would mean a sizeable increase in the capital costs of generation to deliver their plan, pushing up the costs of wind power very significantly. In fact, the costs they assume for renewable electricity generation are three or four times lower than we pay today through Contracts for Difference (CfDs) and Feed-in-Tariffs (FiTs) and are very much lower than achieved in the latest auction round. Using more realistic assumptions about generation costs would double their weighted average generation cost to around £90/MWh.
The RS report calls for 112GW of offshore wind capacity. As discussed above, this is too low to meet their generation target which is also too low. However, their low generation capacity estimate is undeliverable. Extrapolating current offshore development trends shows nearly two thirds of their offshore wind capacity target would remain unbuilt by 2050.
The report then goes on to assume efficiencies and costs for hydrogen electrolysers, storage and generation that do not stand up to scrutiny. The efficiencies are based on high-end projections of what might be achieved by 2050. These would be an enormous stretch to achieve and even if they were, the average of the installed fleet in 2050 is bound to have a lower efficiency. These lower realised efficiencies will push up the costs dramatically. They also assume no leakage of hydrogen stored underground at high pressure for a up to a decade. Assuming some leakage would push up costs further. The individual costings are all based on estimates produced before the latest inflationary surge that has pushed up the costs of everything which means the costs in today’s terms are far too optimistic. Again, even if their costs are achievable by 2050, much of the infrastructure will have to be built using today’s cost base which will push up the average dramatically.
The base-case for their financial assessment assumes a risible 5% cost of capital, lower than the current base rate. Even their sensitivity analysis that uses a 10% cost of capital is probably way too low. Investors are going to require rates of return of 15% or more to invest in immature technologies that will only ever have low load factors. It is likely that using more realistic efficiency estimates and costs of capital will at least double their base case estimates for their proposed hydrogen system.
After all that effort, the system they propose will have a very low energy return on energy invested (EROEI), meaning we will spend more than a quarter of our gross energy to produce the energy we need to live. Throughout human history, we have increased EROEI which has allowed us to thrive. This proposal takes us back to Iron Age times.
Even if their proposed energy system would produce enough to ensure a thriving society and the costs were realistic the report overlooks the human factors that would be required to deliver it. We would need thousands more skilled engineers that would not be available from abroad, because those countries would need them to complete their own projects.
Overall, the report starts with an unrealistically low estimate of future electricity demand which itself is an undesirable outcome. It then goes on to add its own unrealistic claims about the cost and efficiency of hydrogen electrolysers, storage and generation using a risible base case 5% cost of capital. In essence, the report expects us to believe we can deliver lots more renewable energy and a complete hydrogen ecosystem in 2050 for about the same as the cost per MWh of renewables in this year’s renewable auction. It’s a fantasy.
Even if it were deliverable, we would end up with an energy system near the bottom of the energy cliff producing about half the energy we need to run a modern society. It is simply not credible. The extraordinary evidence to support their extraordinary claims simply does not exist. Quite the contrary, there is a plenty of evidence that their claims are a fairy tale. Because the RS report also effectively rules out batteries as the energy storage medium to support intermittent renewables, the entire renewables project should be scrapped.
Introduction
The Royal Society recently released its Large Scale Electricity Storage report authored by Professor Chris Llewellyn-Smith saying we can meet our demand for electricity with wind and solar, supported by large-scale hydrogen storage. They claim that the cost of this approach “compares very favourably” with the cost of low-carbon alternatives. To get to their conclusions, they make some heroic assumptions and make some extraordinary claims about energy demand, generation capacity, the costs of renewables and the cost of storage required to meet net zero targets. This article examines their claims and seeks to find the extraordinary evidence required to validate their assertions.
What is good about the Royal Society Large-Scale Electricity Storage Report
Before diving into the significant weaknesses in the RS report, we should spend a little time focusing on the main positive aspect of the report. The thing that stands out most is the painstaking analysis that has been conducted to understand the very significant changes in the weather that occur on yearly and decadal timescales. They analysed wind and solar records over 37 years to estimate the level of variation we might expect from wind power. They found that we can sometimes have several consecutive years where the wind speed is lower than average. This means that if we are to have a grid powered solely by wind, solar and storage, then we need to build up massive stores of energy in the windy years to be used in the calmer years. They conclude that to consistently deliver their 570TWh of electricity each year, we would need 123TWh of hydrogen storage. Some of that hydrogen may have to be stored for a decade or more before it is used. This has important implications for the economics of storage and effectively rules out batteries as the storage medium. Who would want to spend millions on building a battery or hydrogen storage cavern, even more to fill it and maintain it, yet not see any revenue from it for years after it was completed?
Royal Society Electricity Demand Estimate
Now on to the significant weaknesses. The first thing to look at is the expected demand for electricity in 2050. The report settles on 570TWh as their estimate of demand. This is higher than the 518TWh they claim to be in the 2022 FES report, but lower than the 672TWh in the higher demand scenario of the Government’s 2020 modelling of 2050 electricity demand.
There are several problems with this as a starting point for 2050 electricity demand. First, the 2022 FES report has total electricity demand of 598-810TWh for Residential, Industrial & Commercial and Transport across their Net Zero scenarios for 2050. All these scenarios are higher, some much higher, than the 518TWh assumed in the RS report. These scenarios are much lower than other estimates for a largely nuclear-powered grid in 2050. This report arrived at 1,081TWh based on less optimistic assumptions of the efficiency gains to come from electric vehicles and heat pumps as well as allowing for economic growth and an increase in the population. For reasons discussed below, this estimate may be too low. 1,081TWh is well below the implied 1,338TWh 2050 electricity consumption target suggested by Professor Sir David MacKay set out in Sustainable Energy Without Hot Air (SEWTHA) in 2008. Table 1 below compares scenarios from different sources.
Second, this matters because there is a strong positive correlation between per capita energy consumption and per capita GDP. There are no rich countries with low energy use (see Figure 1).
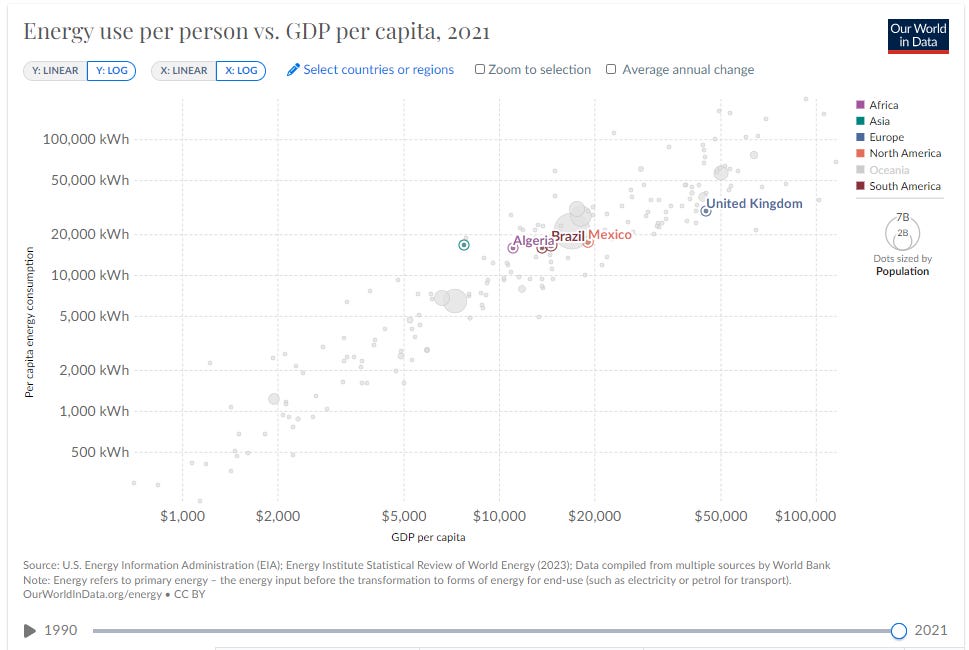
Halving our per capita energy use would put us on a par with countries like Mexico, Brazil, Algeria and Paraguay, all very much poorer countries. To justify halving per capita energy consumption, we need some extraordinary evidence to demonstrate it is a sustainable proposition. Indeed, as Figure 2 (again from Our World in Data) shows there is a strong correlation between reductions in per capita energy consumption and slow growth (or even shrinking economies) on a per capita basis).
To further illustrate the extreme assumptions used to arrive at the overall demand figure, the RS report assumes that we will use only 96TWh of electricity for heat and 119TWh for transport (see p17 of the report). By contrast, according to DUKES Table 4.1.1, domestic consumers and service companies used 410TWh of gas in 2021, the vast majority for heat. Industry used a further 190TWh, probably mostly in industrial processes. Even if we ignore the industrial gas, they appear to be assuming a >4-fold improvement in efficiency from the switch to heat pumps. We might expect heat pumps to perform that well in mild weather, but when it is cold and you need your central heating on, the performance gain will only be around 2.5. It looks like they are planning for us to be cold in winter.
According to DUKES Table 3.2 we used 10,941kt of petrol and 21,228kt of diesel for road transport in 2022. This works out at about 392TWh of energy. The Royal Society is assuming we need only 119TWh of electricity for road transport in 2050, a reduction by a factor of 3.3 assuming no growth in mileage. There is likely to be an improvement in energy efficiency by switching to EVs because electric motors are more efficient than internal combustion engines. However, comparing the official figures for the VW ID3 to diesel and petrol VW Golfs, shows ratio improvements of 2.4 to 3 respectively and that does not include losses in transmission and charging the battery which might be of the order 5-10%. The RS assumptions look more than a little ambitious to say the least.
It looks like the starting assumption of electricity demand is off by a factor of two. This is important, because that means they will need around twice the generating capacity and roughly twice the storage capacity they have estimated. Now let us look at some of the other assumptions.
Unrealistic Profile of Demand
The Royal Society Large Scale Electricity Storage report recognises that demand for electricity varies with the seasons. They use a model from engineering consultancy AFRY to profile the shape of demand on an hour-by-hour basis. The example they show in Figure 3 below (their Figure 1) uses demand from 1992 scaled up to the 570TWh they expect to deliver each year.
As you can see, they expect demand in July to be around 60GW each day and demand in January to be around 33% higher at around 80GW per day. However, by simply using the current electricity demand profile as their template for the future, they have ignored even larger seasonal variations in demand for gas. Of course, we use far more gas in the winter because that is when we have the central heating on. This is illustrated well in one of their references, the H21 North of England report (p15) as seen in Figure 4.
This shows gas demand varying by a factor of five or six from around 480GWh per day (20GW) in summer to 2,400-3,000GWh per day (100-125GW) in winter. Electricity demand varies from around 600GWh per day (25GW) in summer to 960GWh per day (40GW) in winter. Transport demand is relatively stable around 1,440GWh per day (60GW). Total demand is varying from ~105GW in summer to ~210GW in winter or roughly doubling, far more than the 33% increase they assume. The H21 exaggerates the problem somewhat because some of the energy is double counted because some of the gas is also used for electricity generation. However, even after stripping out gas used for that and assuming efficiency improvements from heat pumps and EVs, there will be larger seasonal variations than they have allowed for. This means they will need more wind and hydrogen generation capacity and possibly more electrolyser capacity than they estimate to meet peak demand.
To further illustrate this point, current peak electricity demand in winter is around 45GW. Consider that 24m homes with an average 12kW heat pump operating at a COP of 2.5 on a cold mid-winter night would generate a peak demand of 115GW. That is a total of 160GW without even considering EV charging or the electrification of industrial heat. The AFRY model they use assumes a peak demand of 98.4GW and they plan for 100GW of hydrogen generators to cover it. That is clearly not enough.
Inter-Annual Demand Variation
An additional wrinkle in the RS analysis is that they appear to be assuming that demand is consistent from year to year. Whereas, when domestic and commercial heating are electrified, demand will be much higher in cold years and lower in mild years. This is illustrated in Figure 4 above when the “Beast from the East” pushed up gas demand to around 150GW. Moreover, according to the Met Office Winter 2010 was a very cold year and both 2009 and 2011 were below average. In the RS model, weather conditions meant generation would have been low in the years 2009-2011 consuming most of the store, assuming flat demand (see Figure 5).
It is likely that there was above average heat demand from 2009-11, with exceptional demand in 2010. If variable demand is considered, it is highly likely that the store would have been depleted, implying they need to model for a larger store. They will likely also need more renewable and hydrogen generation capacity to meet the exceptional peak demand. Both these factors will of course increase costs.
Over-Optimistic Electricity Generation Assumptions
To meet their estimate of 570TWh of demand, the RS report says we would need 200GW of installed generation capacity split 80% wind (160GW) and 20% solar (40GW). The wind component would be 70% offshore (112GW) and 30% onshore (48GW). They expect this generation mix to produce on average 741TWh each year. This is more than demand, but because the supply profile of wind and solar do not match demand, storage is required and extra energy must be produced to account for the roundtrip losses in making hydrogen, storing it and then burning it to produce electricity. They assume 89.4GW of electrolyser power to make hydrogen and 100GW of generation capacity to burn it will be necessary. Note that 100GW of hydrogen generation is more than double the current thermal installed generation capacity. They also assume 123TWh of hydrogen storage capacity will be needed as well as possibly compressed air storage which is discussed further below.
Now let us go through the assumptions they have made about the load factors and efficiencies of the different components. They have used load factors for wind and taken from the 2020 BEIS Generation Costs report. Plugging in 57% for offshore wind, 34% for onshore wind and 11% for solar gives the 741TWh average generation they are seeking. However, the estimates of load factors for wind do not bear any resemblance to what has been achieved in practice. According to Energy Trends T6.1, Over the past five years offshore wind has delivered 41.0% and onshore wind 26.4% average load factors. The load factors achieved for solar are slightly below 11% but are close enough to ignore.
Various experts have predicted big increases in load factors for new developments, but these do not seem to have materialised in practice. Moreover, to achieve such high average load factors, the new installations will have to achieve load factors close to the Government’s even more ridiculous higher estimates for 2050 covered here.
Allowing for some technological improvement by assuming the 2050 installed base has average 45% and 30% load factors for offshore and onshore wind respectively, the installed generation capacity would have to increase by more than 20% to ~243GW to meet their 741TWh average target. Even this assumption might be ambitious because there is strong evidence from another GWPF report that load factors decline with age, so the lifetime average may well be lower than this assumption. The load factors above mean 136GW of offshore wind, 58GW of onshore and 49GW of solar would be needed to meet their production target. This would represent a sizeable increase in the capital costs required to deliver their plan. The extra capacity required to deliver the same output will also push up the cost of wind power very significantly (see below).
The other curious thing is that they fail to explain how their planned 40GW of installed solar capacity manages to produce peak output of nearly 80GW on many days in July (see Figure 1). There is clearly some other hidden flaw in their modelling.
[Update 15 Oct 2023]: This anomaly is discussed further in this new article. It appears as though they are being inconsistent in their calculations of generating capacity. [/Update]
Generation Cost Fantasy
The Royal Society Large-Scale Electricity Storage report uses a weighted average of ~£45/MWh (their Figure 24) in 2021 money as their base cost of wind and solar supply (including transmission costs). This compares to the actual results of ~£70/MWh for onshore wind and £63/MWh for solar (both in 2023 money) awarded in AR5. Currently, we pay ~£177/MWh for offshore wind CfDs and £110/MWh for onshore. The average FiT price which is mostly solar was £196/MWh in 2022 with current solar CfDs £106/MWh in 2023 money. Of course, no offshore wind was awarded in AR5, so we have to estimate the costs for projects with near term delivery. The Government’s 2023 estimates of the future Levelised Cost of Energy (LCOE) were taken apart here. In summary, the Government had ignored the increasing costs of turbines and rising interest rates as well as making unrealistic assumptions about load factors. That analysis arrived at a more realistic estimated cost of ~£130/MWh for offshore wind. Even if that estimate was wrong, it is clear that offshore wind costs are well above the ~£55/MWh in 2023 money suggested by the Government in the AR5 auction.
Plainly, the weighted average base generation cost of wind and solar is far higher than estimated in this report. By using the costs of onshore wind and solar in AR5 and estimating £100/MWh for offshore wind (in-line with Aldersey-Williams et al 2019), the weighted average generation cost doubles from their estimate to ~£90/MWh.
Deliverability of Generation Capacity in the Royal Society Large-Scale Electricity Storage Report
Figure 6 below compares the evolution of actual installed capacity of offshore wind, onshore wind and solar to the 2050 targets set in the RS report.
The targets in the RS report call for 112GW of offshore wind capacity shown by the green square which is more than eight times the current 14GW capacity shown by the solid green line. The dotted green line extrapolates the linear trend of installed capacity since 2009. If this trend continues, we might get to ~40GW by 2050, about 72GW short of the target. Even if the sites for these wind farms could be found, it is very unlikely that the rate of installation could be increased to meet this target, especially when one considers that most of the existing fleet will need to be replaced by 2050 too.
With a bit of luck, we might achieve their targets for onshore wind and solar. However, this requires finding sites for these developments and persuading communities to blight their local landscapes and change the use of good agricultural land from food production to electricity production.
However, this generation capacity is only sufficient to meet the relatively meagre annual demand of 570TWh using their optimistic load factors. Using more realistic load factors as discussed above would put offshore wind further beyond reach and make the onshore wind and solar targets much less achievable. To provide the more realistic amount of energy discussed above, the generation capacity will have to at least double to meet 1,081TWh of demand with realistic load factors. There is no chance at all of any of the technologies meeting those targets.
Hydrogen System Costs
Over long timescales, there are large variations in the expected output of wind and solar. This is true on daily, seasonal and multi-annual timescales. To meet demand on a minute-by-minute basis, there needs to be a large storage capacity that can be used to generate dispatchable power. The Royal Society Large Scale Storage report acknowledges there will be a need for very short-term storage in the form of batteries, possibly slightly longer-term storage in the form of Advanced Compressed Air Energy Storage (ACAES) with the vast majority of storage provided by hydrogen in underground caverns. These will be filled in years with above average supply and used up in the leaner years. They have calculated that we will need 123TWh of hydrogen storage capacity to manage these inter-year variations in supply. The calculations supporting this requirement appear to be robust, except for again, they are based upon the low overall electricity demand of 570TWh making no allowance for inter-annual variations in demand. Approximately twice the amount of storage will be required to meet the larger estimate of demand discussed above.
Capex for Hydrogen Components
The capex requirements for electrolysers, storage and generators appear to have been understated. The RS capex estimates for electrolysers of $450/kW in 2050 are at the low end of IEA predictions for 2050 of $200-900/kW. They are also far lower than the current costs of $1,100-1,800/kWh that the IEA estimates. The IEA predictions for 2050 are based on a study from 2018 which is well before the recent inflationary wave and increase in interest rates. It is therefore likely that the estimated capex requirement for the electrolysers is far too low. Even if those cost estimates are met, the average cost of the fleet in 2050 is bound to be much higher.
Similarly, the H21 report used as the source for storage capex is from 2018 so again does not factor in the inflationary surge since 2020.
The RS report assumed £315/kWe (~$400/kWe) capex for four-stroke hydrogen-fired electricity generation is much lower than that achieved in two of the non-hydrogen projects it cites. The 76MW Goodman Energy Centre project in Kansas cost $62m which gives a cost of $816/kWe in 2008 dollars, more than twice the estimate in the RS report. After the recent inflationary surge and even allowing for some technological improvement, these costs are likely higher in 2023 money. Moreover, the 2014 600MW IPP3 project in Jordan cost $800m or $1,333/kW, more than three times the RS estimate.
It is obvious that the capex estimates in the report are far too optimistic.
Hydrogen System Efficiency
There are also significant concerns about the efficiency assumptions for the electrolysers and 4-stroke engines they plan to use to make the hydrogen and generate electricity. They assume 74% efficiency for the electrolysers. The RS report itself says the efficiency of Polymer Electrolyte Membrane (PEM) electrolysers is currently 40-67% (IRENA) or 55-60% (IEA). IRENA predicts electrolysers to hit >74% efficiency by 2050 and IEA expects 67-74% efficiency by that date. It is foolhardy to expect the full 89GW of electrolyser capacity to achieve the top-end efficiency estimates in 2050. First, these are just projections, not actual measured results and second, the fleet will have to be built over a prolonged period of time so even if the units installed in 2050 achieve the projections, the average efficiency is bound to be lower. Moreover, powering electrolysers using intermittent energy degrades their performance which will lead to lower efficiencies and probably shorter useful lives. Assuming more realistic efficiencies and shorter operating lives will increase the size of fleet required and of course significantly increase the capex and unit cost of hydrogen produced.
The RS report also assumes the generators producing electricity from the stored hydrogen is 55%. This derived from a projection published by McKinsey that shows the maximum expected efficiency of such four-stroke engines might be 55% at close to full load. However, this 2023 study suggests that a 12l V12 high-compression hydrogen engine for use in locomotives might achieve a peak efficiency of 46%. The Kansas project discussed above indicates an efficiency of 44.2%. It is unlikely that the generators will be operating at optimum load conditions at all times because they will have to vary their output according to changes in demand. Again, it seems overly optimistic to assume 55% efficiency based on just one projection when others show significantly lower results. More realistic efficiency assumptions will lead to higher costs because more hydrogen will be needed to produce the required amount of electrical energy.
Finally, they plan to store the hydrogen in underground salt caverns at 300bar and assume such caverns are gas tight. This is already carried out on a small scale for short-term storage of hydrogen at chemical plants. However, hydrogen is a tiny molecule that can diffuse through most things. Even if such a cavern is gas-tight for methane or air, it is difficult to believe that such caverns will be able to seal hydrogen at such high pressures for up to a decade without some leakage. This study from Gaffney Cline suggests leakage rates in the range 2-18%. However, the period over which that leakage is measured is not clear. They do suggest that the risk of leakage from salt caverns is lower than from other rocks such as depleted gas fields.
If there is any leakage at all, and there is bound to be, that will reduce overall system efficiency and lead to increased costs as well as potential safety concerns.
Cost of Capital
Throughout the report, they assume a default cost of capital of 5%. With the Bank of England base rate currently sitting at 5.25%, this is a risible assumption. They also discuss the impact of a 10% cost of capital. This increase in interest rates leads to the costs of making, storing and burning hydrogen going up by ~50% and pushes up the cost of ACAES significantly.
The technologies to be used in the proposed electrolysers, storage facilities and generators is immature and the planned load factors are very low. Therefore, even a 10% cost of capital is probably not enough to compensate for the level of risk being taken. An estimate of 11% current cost of capital for offshore wind was derived from the premium over long bond rates in the Government’s different Generation Cost reports. Offshore wind is a relatively mature technology whereas these hydrogen technologies are not. It would not be surprising to see investors demanding returns of 15% or more before supporting such projects. Of course, this would push the costs up even more dramatically, probably more than doubling their estimates at 5% cost of capital.
Of course, increased interest rates also push up the costs of wind and solar generation, but they have already been accounted for in the discussion above.
System EROEI
One thing missing from the Royal Society Large Scale Electricity Storage report is any discussion about Energy Return on Energy Invested (EROEI). The importance of EROEI is discussed here. Weissbach calculated the EROEI of various generation technologies in his seminal paper from 2014. Wind without backup had an EROEI of ~16 and solar at German latitudes 3.9. He then adjusted these figures to take account of the buffering or storage required to enable these technologies to deliver power on demand. The buffered EROEI of wind and solar was calculated at 3.9 and 1.6 respectively. The buffering technology he used was hydro power. There is no data specifically about the EROEI of wind and solar buffered by hydrogen. There is reason to believe it maybe even lower than Weissbach calculated because the embedded energy in 89GW of electrolyser capacity, 123TWh of storage caverns and 100GW of hydrogen-fuelled generation capacity will be very large and these systems will not generate any more net energy from the primary wind and solar sources.
Using Weissbach’s calculated buffered EROEI figures for wind and solar, the weighted average EROEI for the whole system is around 3.8. This and estimates of the EROEI of the grid in 1998, 2008 and 2021 as well as the Leading the Way scenario in the latest National Grid FES report and the nuclear dominated plan for 2050 are plotted on Figure 7 below.
As can be seen, the Royal Society plan takes us a very long way down the energy cliff, where we are spending more than a quarter of our gross energy to produce the net energy we need to live. This is well below the economic threshold of seven assumed by Weissbach. This alone should consign the Royal Society plan to the dustbin of history.
[Update 10 Dec 2023:] This new article attempts to calculate the EROEI of this system from first principles concludes that the EROEI will be in the range 7.5-8.5, which is better than using Weissbach data but still an undesirable outcome [/Update]
Overall Costs Understated
Section 8 of the report pulls it all together and arrives at estimated overall system costs of electricity in 2050 using wind, solar and largely hydrogen storage supplemented by 15GWh of battery storage to maintain grid stability as shown in Figure 8 (their Figure 24).
This results in an estimate of the full system costs of electricity at ~£52-62/MWh with a 5% cost of capital and the weighted average cost of base wind and solar generation in 2021 prices of £30.20/MWh. At the top end of estimates, full system costs are in the range ~£78-92/MWh with a 10% cost of capital and base generation costs of £45/MWh. The 10% cost of capital does not seem to have been applied to the weighted average cost of the base renewable power.
This report essentially says the full system cost of electricity including all the base renewable generation, hydrogen electrolysis, transport, storage, backup generation, transmission and batteries will be less than the costs achieved in AR5 in most scenarios and in most cases, cheaper than gas-fired generation today. It is a fantasy.
The models they use are very complex and it is too difficult to propagate more realistic assumptions on extra capacity, increased capex, lower load factors, higher leakage rates, lower efficiencies and higher interest rates throughout. Realistic costs of the base renewable generation would double the starting baseline of £45/MWh and more realistic assumptions about the true cost of hydrogen production, storage and generation would likely at least double too. It is not too much of an exaggeration to say the actual costs would be at least double the numbers they estimate for a 10% cost of capital and £45/MWh renewable generation. This would put the total system costs over £180/MWh.
[Update 10 Dec 2023:] The impact of more realistic costs for the hydrogen system is discussed this new article which concludes the realistic costs are likely well over £200/MWh and maybe even over £250/MWh. [/Update]
Even that would be undeliverable because there is very little chance of expanding offshore wind generation at the rate required.
Human Factors
Aside from the technical and financial flaws in the Royal Society report another set of considerable difficulties need to be overcome, namely the human resources required to deliver the engineering reality. Each billion pounds of project costs will require approximately 1,000 person years of professional engineering time. Most of these engineers will have to be home produced because we cannot take them from their home countries where they will be needed to reach their own net zero targets. The materials requirements for the global net zero project will require more than a tenfold increase in the mining of key materials (including the rare earth metals) requiring even more professional engineers. The sequencing of the projects is critical, as this extra electricity needs to be available at least as fast as the deployment of electric vehicles and heat pumps. Finally, all this needs buy-in from the public, and for a start, the planning delays will thwart even the start of the projects.
Conclusions
The starting point for the Royal Society Large-Scale Electricity report is Government and NGO plans for an energy scarce world in 2050. The evidence from Our World in Data shows this will likely lead to lower GDP per capita and thus lower living standards which is clearly an undesirable outcome. This base assumption is then buttressed by flaky Government estimates of the future cost of renewable energy. These estimates are based on unrealistic assumptions of turbine costs, load factors and the costs of capital.
The report then adds its own unrealistic assumptions about the cost and efficiency of electrolysers, storage and generation technologies and its base case uses a risible 5% cost of capital. Even their 10% cost of capital sensitivity analysis is probably too low. In essence the report expects us to believe we can deliver lots more renewable energy and a complete hydrogen ecosystem in 2050 for about the same as the cost per MWh of renewables in this year’s AR5.
Even if it were deliverable, we would end up with an energy system near the bottom of the energy cliff producing about half the energy we need to run a modern society. It is simply not credible.
The RS report shows the dangers of “official” figures propagating from one institution to another in a daisy chain. The thing is daisy chains are pretty but very fragile. Hopefully, this article has exposed that fragility and demonstrated the extraordinary evidence required to support their extraordinary claims is simply not there. Quite the contrary, there is a plenty of evidence that their claims are a fairy tale. The Royal Society report shows that their vision of 2050 does not quite violate the first law of thermodynamics, but it goes on to break all the basic tenets of sound engineering. Because the RS report also effectively rules out batteries as the energy storage medium to support intermittent renewables, the entire renewables project should be scrapped.
If you have enjoyed this article then please share it with your family, friends and colleagues and sign up to receive more content.
It's worse than we thought, Jim!
Your comparison with Algeria, Mexico, Brazil and Paraguay ignores the fact that they are rather warmer countries than the UK, with relatively little need for heating (and also limited aircon use). If we correct for that the result is a much lower standard of living than those countries.
I remember the blocking anti-cyclone of 2010 which becalmed the entire country for about a month. That didn’t matter much to the electricity supply as at that time our dependency on weather-dependent wind power was low.
It was also bitterly cold midwinter when power is needed most and the roads were all salted. I had to journey from Nairn to Glasgow by car but my windscreen skoosher was frozen solid and I had to stop every few miles to clean the windscreen of road salt manually.