Introduction
Regular readers will know that I have written a number of articles that cover the importance of Energy Return on Energy Invested (EROEI, sometimes abbreviated to EROI) and its close cousin the Net Energy Cliff.
I have often wondered about the economic impact of sliding down the Net Energy Cliff and this article is my first attempt at quantifying the scale of the problem. To do this, I have introduced a new concept that I am calling the Gross Energy Mountain which is essentially the inverse of the Net Energy Cliff and identifies the extra gross energy required to produce the net energy we need to run society as EROEI falls. This cost of this extra energy can be quantified and we can consider the impact on society. This article represents my first thoughts on this and depending upon the feedback, it may lead to further articles exploring the concept further.
What is EROEI?
Starting with the basics, what is EROEI? In simple terms, EROEI is the ratio of useful energy out over the energy invested to get it. The energy invested includes the energy required to extract fuels like natural gas from the ground. It also includes the energy required to extract, refine and process the minerals required to make things like solar panels and wind turbines. It also includes the embedded energy in necessary infrastructure such as the factories required to make solar panels and the pipework to transport natural gas. In his work, Weissbach produced a chart of the EROEI of many different electricity generating technologies (see Figure 1).
The blue bars represent the raw EROEI figures for each technology. The yellow bars recognise that some technologies are intermittent and their output needs to be “buffered” with some sort of storage that itself includes embedded energy. The resulting “buffered” EROEI is a real-world estimate of the performance of intermittent technologies.
What is the Net Energy Cliff?
The reason EROEI is important is that if we spend too much time and energy gathering energy then we have much less time and energy available for other activities and society starts to degrade. This is best illustrated by the “Net Energy Cliff” described by Euan Mearns (see Figure 2).
Net Energy is the surplus energy available to society after deducting the energy used in energy gathering activities. If we have EROEI = 1, then net energy is zero. We are using as much energy to gather energy as the useful energy produced. If EROEI is less than 1, then we have an energy sink. It is possible to plot net energy as a percentage of total energy against EROEI. The blue area on the chart shows how the energy left for society varies with EROEI. As can be seen, when EROEI starts to get into single figures the energy available for society starts to plummet rapidly until it reaches zero when EROEI reaches 1. Note that the Leading the Way scenario in the National Grid ESO Future Energy Scenarios Report (FES) implied a system EROEI of around 10.7 as shown in in Figure 3.
Recent publications have advocated that we run society using solely wind and solar power, such as the Royal Society report on large scale storage and a report from Oxford University. Both would likely result in a much lower overall EROEI, once buffering or storage is taken into account.
What is the Gross Energy Mountain?
If we turn the Net Energy Cliff around, we can think of the extra energy required to make the net energy society needs as EROEI varied. Then we get the Gross Energy Mountain as shown in Figure 4.
When EROEI is around 30, the total gross energy we need to make 100 units of net energy used by society is about 103. If EROEI falls to 20, then the total gross energy rises to 105, not a very big move. However, if EROEI falls to 10, then the gross energy required rises to 111, or 117 when EROEI falls to 7, rising eventually to 200 when EROEI falls to 2. This shows there is a non-linear relationship between falling EROEI and gross energy requirements. At first, the increase is barely noticeable, but as EROEI falls further, suddenly we have a mountain to climb to produce the net energy we need to run society.
This extra energy required as EROEI falls can also be expressed as a percentage of net energy as shown in Figure 5.
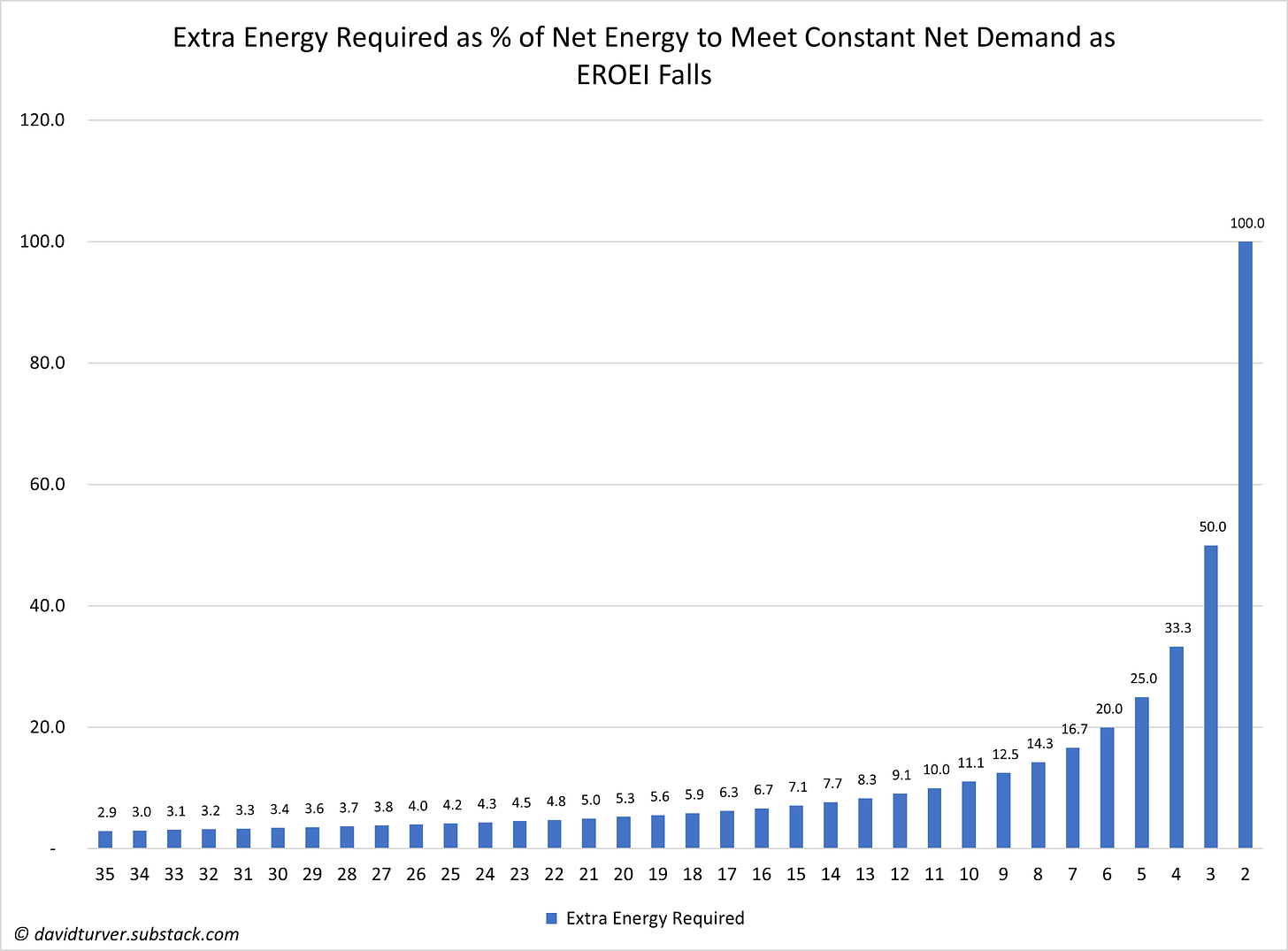
This is why claims of efficiency gains from electrification using renewables are so dangerous. They do not take account of the extra energy required to generate the electricity from low EROEI renewables. This extra energy required acts as a hidden tax on the economy.
Economic Impact of Low EROEI
Now we have the base energy data, we can consider the potential economic impact of reducing EROEI depending upon the scale of final electricity demand. Starting with the energy impact, we can calculate the extra TWh of gross energy required to meet a certain level of final demand for different EROEI values. The extra gross energy required as EROEI moves from 30 down to 20, 10, 7 and 3 can also be calculated as shown in Figure 6 below. I have chosen three different final demand or net energy requirements for 2050. The Royal Society report on large scale storage assumed final demand of 570TWh. My own estimate of final demand, making less optimistic assumptions about the efficiency gains from electric vehicles (EVs) and heat pumps and allowing for an increase in population and economic growth was ~1,100TWh. A recent report from Oxford University suggested final demand would be 1,500TWh, after allowing for the growth of some energy intensive industries such as cultivated meat.
As can be seen if EROEI moves from 30 to 20, for a final demand of 1,500TWh, the extra gross energy required is 27TWh, which is a tiny proportion of net energy demand and so would be barely noticeable. However, as EROEI falls further, to the levels we might expect from buffered wind and solar renewables, then the extra energy required rises substantially and becomes increasingly significant.
Now we have the energy impact, we can make some calculations of the economic impact. The extra energy must come from somewhere, and if our net demand is fixed, then the extra gross energy must be imported. Most likely, it will be imported in the form of embedded energy in imported goods. As most primary energy today comes from coal, gas or oil, I have converted the extra gross energy to barrels of oil equivalent (at 1,700kWh per barrel) and assumed $80/barrel and GBP/USD = $1.225. The cost of this extra imported energy can then be calculated and is shown in Figure 7 below.
As can be seen, if net energy demand is 1,500TWh, the extra cost of energy rises by about £1bn as EROEI falls from 30 to 20. However, as EROEI falls further, the cost of the extra gross energy rises to £4.4bn if it falls to 10 and £7.6bn if it falls to 7. These numbers compare to the trade deficit in 2022 of £68bn (or about £30bn in the pre-Covid years). As can be seen, these numbers are very significant when compared to the overall trade deficit. If we continue down the path of a lower EROEI energy system, our trade deficit would widen significantly.
Conclusions
We have established that EROEI and the net energy cliff are important concepts that illustrate the dangers of reducing the EROEI of our energy system. We have also established that there is a significant strand of opinion in academic circles that advocate for a renewables only solution to our growing electricity needs. Once the need for storage or buffering has been considered to match supply and demand, then the EROEI of our energy system will fall further sending us scrambling down the net energy cliff.
If we turn around the net energy cliff and instead look at the extra gross energy required to generate the net energy we need to run society, we can clearly see the gross energy mountain we would have to climb to realise the dreams of renewables advocates.
That extra energy will have to be imported as embedded energy in goods we bring in from abroad. By converting that energy to oil equivalent, we can see that as EROEI falls, this imported embedded energy would significantly increase our trade deficit, leading to even more deindustrialisation and associated social decay. Advocates of renewables should be careful what they wish for.
If you have enjoyed this article, please share it with your family, friends and colleagues and sign up to receive more content using the buttons below.
Please keep writing about EROEI. Something I would like to see discussed is, 'What might happen if we get energy from sources that have a absurdly high EROEI?' Dual Fluid, a company proposing a 5th generation nuclear reactor that uses a molten uranium core cooled with molten lead suggests they will achieve an EROEI of 1000.
That might be high. But if we can reprocess spent fuel and use it in breeder reactors there's probably enough Uranium to last for 1,000 years.